PULSE
POWER PERFORMANCE OF
ELECTROCHEMICAL CAPACITORS:
TECHNICAL
STATUS OF PRESENT COMMERCIAL DEVICES
John R. Miller
JME, Inc.
17210 Parkland Drive
Shaker Heights, Ohio 44120 USA
Phone/Fax 216-751-9537
Presented at the "8th International Seminar on Double Layer
Capacitors and Similar Energy Storage Devices", Deerfield Beach; Florida, December 7-9, 1998.
ABSTRACT
In recent years, applications have developed where
a high-power electro-chemical capacitor (EC) could provide benefits to a power system. Capacitor
manufacturers and developers have responded to these emerging markets with EC products having
enhanced power performance. Presently, it is difficult to determine the performance capability
of such capacitors based on manufacturer specifications and data sheets because test procedures are not
defined, incomplete, or inconsistent. This paper presents pulse power performance information
of commercial or near commercial electrochemical capacitors measured using a consistent test method.
Measurements are reported on seventeen capacitors obtained from nine different manufacturers. Testing
was performed at 25 C on all samples. Three samples showing the best pulse power performance were
also tested at 0, -20, and -40 C. A new data analysis approach was developed to help quantify
capacitor pulse power performance. A pulse power figure-of-merit is defined. The developed approach
is more general than Ragone plots and has great applicability for pulse power applications.
INTRODUCTION
Energy storage in the electric double layer, created at
the interface between an electrode and an electrolyte, was recognized more than a century ago. However,
products based on this energy storage concept have appeared only in the last 20 years. Initially they
were developed to meet the backup power requirements of volatile computer memories. Several companies,
primarily Japanese, have commercialized such ECs. They range in size from a fraction of one farad
up to 50 F (up to tens of Joules of stored energy) with working voltages up to about 5.5 V. For these
applications, ECs have been optimized for high energy density, low self-discharge rate, and low
cost. By design, such devices have relatively high equivalent series resistance (ESR), in the range
of tenths to tens of ohms, and thus offer relatively low power performance. Most of these products are
not well suited for pulse power applications.
More recently, ECs have been developed for truck and
locomotive engine starting [1,2], for electric and hybrid vehicles for load power leveling [3,4],
for use with batteries in digital wireless communication applications [5,6,7], for energy storage in UPS
systems [8], and for electric vehicle traction [9]. Each of these applications requires an optimization
different from earlier products. For example, internal combustion engine starting using ECs alone, or
in combination with starting, lighting, and ignition (SLI) batteries, requires very high current delivery
for a period of up to a few seconds. These ECs are optimized for energy delivery on a time scale
of about 1 second. And for digital communication applications, the EC must be optimized to efficiently
deliver pulse power having duration of 0.5 ms and a period of 5 ms. These capacitors also must have
high cycle-life, small size, and low cost.
There are many types of batteries on the market today.
That is because each offers one or more unique advantages, for instance small size, high cycle-life,
efficient pulse power performance, fast recharge rate, or low cost. As with battery technology,
each EC manufacturer has optimized its products for specific applications. Consequently, one technology
will not be universally optimum for all applications. Thus, some of the EC products on the market or
about to be introduced should be better suited than others for pulse power operation.
This study focuses on measuring commercial or near commercial
electrochemical capacitors to identify those technologies having superior pulse power performance. This
investigation was limited to low voltage devices, voltages considered important for digital wireless
communication. The present work updates and greatly expands EC product
performance information reported by Nishono in 1993 [10] and by Miller in 1995 [11].
CAPACITOR PRODUCTS
Seventeen capacitors obtained from nine different manufacturers
were evaluated in this study. Many of these capacitors were samples provided specifically for the study.
They represent commercial ECs and those near commercialization. In the following product summary,
capacitor dimensions do not include electrical leads or terminations. Weights do include all coverings
(shrink wrap and/or labels) and terminations. Product information follows.
CAP-XX
Five different capacitors were evaluated, each prismatic
in form factor and rated at 2.5 V. The largest was 250 F, dimensions of 190 x 110 x 18 mm with
a mass of 295 g. The second was 120 F, and 50 x 50 x 11 mm with a mass of 26 g. The third was 30
F with a size of 50 x 50 x 10 mm with a mass of 25 g. The fourth was 10 F and 50 x 50 x 5 mm with
a mass of 17 g. And the fifth EC was rated at 0.3 F and the size of a plastic credit card (85 mm
x 53 mm) and 2 mm thick. It had a mass of 12 g.
ECOND
Two of their products were evaluated, each rated at
16 V and storing ~8 kJ of energy. The 8/16/0.8 model was a right cylinder 226 mm in diameter and
82 mm tall. Its mass was 9.6 kg. The second model was a 8/16/10 that was 226 mm in diameter and 135
mm tall. Its mass was 13.4 kg.
ELIT
Two of their products were evaluated. Both were prismatic
in design. The first was a 9.4 F, 15 V device having dimensions in cm of 17 x 17 x 5 and a mass of 4.8 kg. The
second was a 20 kJ, 13 V capacitor with dimensions in cm of 17 x 17 x 22.5 and a mass of 15.3 kg.
ELNA
The product evaluated was a 50 F, 2.5 V rating EC with
a form factor of a right cylinder 26 mm in diameter and 40 mm long. Its mass was 24 g.
ESMA
Their product was prismatic in design, rated at 13 V,
and had dimensions in cm of 28.5 x 6.4 x 14 and a mass of 5.1 kg.
Maxwell
Three different ECs were evaluated, each having a prismatic
form factor. The largest was the PC7223 model, a 2700 F, 2.3 V EC with dimensions of 164 x 62
x 62 mm and a mass of 830 g. The second was a PC0323, 100 F, 2.3 V capacitor with dimensions of 33.6
x 17.1 x 53 mm and a mass of 34 g. The third was a PC223D 8 F, 2.3 V rated EC having dimensions
of 23 x 4.3 x 29 mm and a mass of 6.3 g.
Panasonic
The product evaluated was rated at 800 F and 2.3 V. The form
factor was a right cylinder with a diameter of 55 mm and a height of 125 mm. The mass was 320 g.
Powercell
Their product was a 20 F, 30 V rating capacitor with
a prismatic form factor with dimensions in cm of 15.4 x 15.4 x 3.2. Its mass was 1047 g.
Powerstor
The product evaluated was a 1.6 F, 2.5 V rated capacitor
having a right cylinder form factor 11.2 mm in diameter and 30.5 mm long. Its mass was 4.4 g.

CAPACITOR MEASUREMENTS
Prior characterization standards have been limited in
scope and are inadequate to properly characterize the pulse power performance of an EC. For instance,
the DOD-C-29501 test procedure measures capacitor ESR and capacitance using methods only important
for low-rate capacitors. These include ESR measurements at exactly 1 kHz and charging capacitance
measurements at approximately a one-minute rate. Power performance is not addressed.
The DOE "Electric Vehicle Capacitor Test Procedure
Manual" [12] is more suited for characterizing power performance of ECs. But it has limitations
in determining pulse power performance. For instance, this procedure specifies capacitor charge/discharge
measurements in times of 2 seconds or longer (Procedure 1). Thus, it does not provide information on the
desired sub-second pulse power performance.
Yet another method for measuring the power performance
of an EC involves developing so-called Ragone plots of energy density versus power density. With this
approach, a capacitor is typically discharged at constant power from its rated voltage to one-half
this voltage, and the energy that is delivered during the discharge is recorded and plotted against
the value of the discharge power. Reduced units are usually used. This approach has limited practical
value because few applications involve discharging a capacitor at constant power to one-half its rated
voltage. Most applications have a more complicated power profile. Many involve a pulsed discharge
that results in an extremely small change in capacitor voltage, for instance, capacitors used in combination
with batteries for digital communication.
In this study, a new approach was developed to assess and
compare pulse power performance of ECs. It is based on complex impedance measurements. This approach
involves identifying the frequency fo at which a capacitor's impedance reaches a -45 degree
phase angle then taking the reciprocal of this frequency to obtain a characteristic capacitor
response time To. The value of the imaginary part of the impedance Z'' at fo is then
used to calculate available capacitor energy using the equation Eo = 1/2 C V2,
where C = -1/(2 p fo Z'') and V is the
rated voltage of the capacitor. Finally, available energy per mass Eo/M and energy per volume
Eo/v are plotted against response time To. This approach is very general and useful for
determining the power performance of electrochemical capacitors in situations other than constant-power
discharge. It allows a direct quantitative comparison between technologies and products.
The deficiencies of using a simple series-RC circuit
to examine the power performance of an EC has been previously discussed [13]. In brief, a series-RC
equivalent circuit model is inadequate to describe the EC's response under moderate to high-rate discharges
because of the distributed charge storage. In general, EC performance is poorly represented by such a
simple one-time-constant model. And this simple series-RC model becomes most problematic at high
power levels, those important for pulse power applications. The approach in this study does not have such
shortcomings. It makes no model assumptions but rather uses direct
measurements to determine the available capacitor energy at a characteristic response time defined
by the frequency where resistance and reactance are equal (-45o phase angle).
For help in comparing the pulse power performance of different
technologies and products, two figures-of-merit (FOM) were formulated based on the described measurements.
These are defined as the available gravimetric energy density Eo/M divided by the EC's
characteristic response time To, and available volumetric energy density Eo/v divided
by the EC's characteristic response time To. Thus, FOM units are gravimetric and volumetric
power density, i.e. watts per gram and watts per cm3. ECs with the same FOM should be
equally suited to meet the electrical performance requirements in a given pulsed power application.
ECs having the largest FOM values will form the smallest or the
lightest systems. ECs with the same volumetric FOM should be comparable in size for a given application. This
assumes that the volumetric performance of the EC is maintained with scaling, which should be true for devices
having prismatic form factors. ECs with the same gravimetric FOM should be comparable in mass for a given
application. This assumes that the gravimetric performance of the EC is maintained with scaling.
Capacitors in this study were first weighed and measured for
physical dimensions. Then their impedance was measured using a Solartron model 1250 frequency response
analyzer with an EG&G PAR model 273 potentiostat. The amplitude of the applied ac signal was 5 mV
for all capacitors. The measurement range was 65 kHz to 1 mHz, and for some samples down to 100
mHz. Ten measurements per frequency decade were
measured. Bias voltage was at approximately 80% of the rated voltage of each capacitor except for capacitors
with voltage ratings above 10 V. In such situations, the bias was 8 V.
All capacitors were measured at room temperature and
the three showing the best pulse power performance were also measured at 0, -20, and -40 C. For impedance
measurements below room temperature, each capacitor was maintained in a forced-air environmental chamber
at bias voltage until thermal equilibration. Chamber residence times depended on the capacitor mass,
but were at least one hour at temperature before testing started.

TEST RESULTS AND ANALYSIS
Figure 1 shows Bode plots of typical results for
six of the capacitors at 25 C. Note the non-ideal nature of some of the devices in the magnitude
of the impedance |Z| and in the phase angle. The intersection of the data with the horizontal
line drawn at -45 degrees identifies fo, which is used to obtain the capacitor response
time To = 1/ fo. The value of the imaginary part of the impedance Z'' at
fo is then used to calculate available capacitor energy using the equation Eo =
0.5 C V2 where C = -1/(2 p
fo Z'') and V is the rated voltage of the capacitor. Finally, available
energy per mass Eo/M and energy per volume Eo/v are calculated.
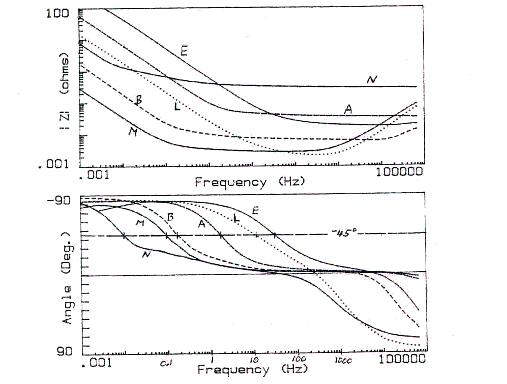
Figure 1: Bode plots for six of the capacitors at
25 C. The key is given in Table I. Note the non-ideal characteristics of most of these capacitors.
Table I summarizes the test results at 25 C. The
letter in the left column is keyed to data points in the figures. Capacitor volumes used in the
calculations do not include electrical leads or terminations. Capacitor masses used in the
calculations include all coverings (shrink wrap and/or labels) and terminations.
TABLE I: Test results at 25 C for the seventeen
capacitors evaluated in this study. The response time is determined as the reciprocal of the frequency
at which the impedance reaches -45o phase angle. Energy is derived from the impedance value
at that same frequency. The FOMs are equal to the gravimetric or volumetric energy density
divided by the response time.
|
EC PRODUCT |
E/M
(J/g) |
E/v
(J/cm3) |
Response
time (s) |
Mass FOM
(W/g) |
Volume FOM
(W/cm3 ) |
A |
Powerstor |
1.3 |
1.9 |
0.63 |
2.1 |
3.0 |
B |
Maxwell PC0323 |
6.2 |
6.9 |
6.3 |
1.0 |
1.1 |
C |
Maxwell PC7223 |
9.7 |
12.8 |
20 |
0.5 |
0.64 |
D |
Maxwell PC0223 |
4.5 |
9.9 |
9.1 |
0.5 |
1.1 |
E |
CAP-XX card |
0.047 |
0.062 |
0.037 |
1.3 |
1.7 |
F |
CAP-XX 120 F |
7.4 |
7.0 |
10 |
0.74 |
0.7 |
G |
CAP-XX 30 F |
1.3 |
1.3 |
0.48 |
2.7 |
2.7 |
H |
CAP-XX 10 F |
0.54 |
0.61 |
0.15 |
3.6 |
4.1 |
I |
CAP-XX 250 F |
2.2 |
1.6 |
1.0 |
2.2 |
1.6 |
J |
ELNA |
4.4 |
5.0 |
12 |
0.36 |
0.4 |
K |
Panasonic |
6.7 |
7.2 |
20 |
0.34 |
0.37 |
L |
ELIT 9.4 F |
0.093 |
0.31 |
0.097 |
0.95 |
3.2 |
M |
ESMA |
2.2 |
4.3 |
10 |
0.22 |
0.43 |
N |
Powercell |
7.3 |
10 |
110 |
0.07 |
0.09 |
O |
ELIT 20 kJ |
1.2 |
2.7 |
5.9 |
0.2 |
0.46 |
P |
ECOND 8/16/0.8 |
0.68 |
2.1 |
7.7 |
0.09 |
0.27 |
Q |
ECOND 8/16/10 |
0.51 |
1.7 |
1.2 |
0.43 |
1.4 |
Volumetric energy density from the above table is plotted against
response time in Figure 2. Labels on the graph correspond to the left column in the table. Constant FOM
values are shown. Again, devices having the largest FOM are best suited for pulse power applications.
Gravimetric energy density versus EC response time is shown
in Figure 3. Constant value FOM Lines are also shown.
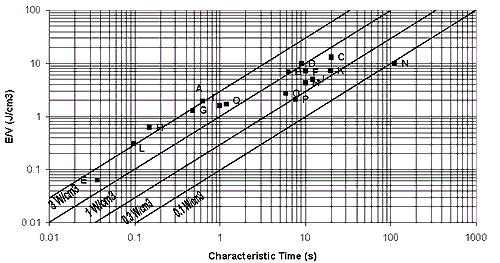
Figure 2: Volumetric energy density versus
response time. Data points are keyed to Table I.
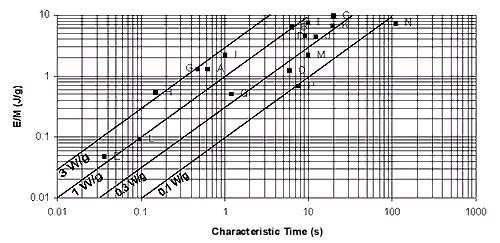
Figure 3: Gravimetric energy density versus
capacitor response time. The data points are keyed to Table I.
Table II summarizes the test results at 25, 0,
-20, and -40 C for three of the capacitors that showed the highest volumetric FOM, indicating
the best pulse power performance.

TABLE II: Test results for three of the capacitors
evaluated in this study at four temperatures.
EC PRODUCT
Temperature |
E/M
(J/g) |
E/v
(J/cm3) |
Response
time (s) |
Mass FOM
(W/g) |
Volume FOM
(W/cm3 ) |
Powerstor 25 C |
1.3 |
1.9 |
0.63 |
2.1 |
3.0 |
0 C |
1.2 |
1.8 |
1.0 |
1.2 |
1.8 |
-20 C |
1.1 |
1.6 |
1.9 |
0.6 |
0.8 |
-40 C |
0.9 |
1.4 |
14 |
0.06 |
0.1 |
CAP-XX 10
F 25 C |
0.54 |
0.61 |
0.15 |
3.6 |
4.1 |
0 C |
0.38 |
0.43 |
0.14 |
2.7 |
3.1 |
-20 C |
0.26 |
0.29 |
0.15 |
1.7 |
1.9 |
-40 C |
0.12 |
0.13 |
0.10 |
1.2 |
1.3 |
ELIT 9.4
F 25 C |
0.093 |
0.31 |
0.097 |
0.95 |
3.2 |
0 C |
0.086 |
0.29 |
0.12 |
0.70 |
2.4 |
-20 C |
0.081 |
0.27 |
0.19 |
0.42 |
1.4 |
-40 C |
0.079 |
0.26 |
0.48 |
0.17 |
0.6 |
DISCUSSION
Using the new approach described for examining EC pulse
power performance, major differences are observed among the seventeen capacitors examined in this
study. Several offer both gravimetric and volumetric pulse power advantages over the other ECs tested.
Some offer advantages in either gravimetric or volumetric pulse power performance.
Referring to the energy density versus response time plot
in Figure 2, EC products appear to be divided into several groups, perhaps because of common materials
or designs. ECs offering the best volumetric pulse power performance are clearly evident.
The groupings in Figure 3 are not as tight as in Figure 2 but they
nevertheless clearly show gravimetric pulse power performance advantages for several ECs.
Table II listed temperature effects on pulse power performance
for three ECs. Discernible differences are evident among the products, which may be important in some
applications.
ACKNOWLEDGMENTS
The author would like to thank the EC manufacturers
who provided samples, which made this study possible.
REFERENCES
- A. Beliakov, "Russian Supercapacitors to Start Engines,"
Battery International, p102, (April 1993); A.I. Beliakov, "Investigation and Developing of Double Layer Capacitors
for Start of Internal Combustion Engines and of Accelerating Systems of Hybrid Electric Drive," Proc. 6th
Int. Seminar on Double Layer Capacitors and Similar Energy Storage Devices, Deerfield Beach, FL (Dec.
9-11, 1996).
- A. Ivanov, A. Gerasimov, and A. Vishnevshy,
"ECOND Double-Layer Super-High-Energy Capacitor for Pulse Power Applications," Proc.
3rd Int. Seminar on Double Layer Capacitors and Similar Energy Storage Devices, Deerfield Beach,
FL (Dec. 6-8, 1993).`
- A.F. Burke, J.E. Hardin, and E.J. Dowgiallo, "Applications of
Ultracapacitors in Electric Vehicle Propulsion Systems," 34th Power Sources Conf, Cherryhill,
NJ (June 1990).
- L.A. Viterna, "Hybrid Electric Transit Bus,", Proc. SAE Int. Truck
and Bus Meeting and Exposition, paper 973202, Cleveland, OH (Nov. 17-19, 1997).
- J.R. Miller, "Battery-Capacitor Power Source for Digital
Communication- Simulations Using Advanced Electrochemical Capacitors", Electrochem.
Society Extended Abstract 66, Vol. 95-2, Chicago, IL (Oct. 8-13, 1995).
- J.R. Miller, "Capacitor-Battery Power Sources: Designing for
Optimal Performance," Proc. Fifth International Seminar on Double Layer Capacitors
and Similar Energy Storage Devices, Boca Raton, Florida (December 4-6, 1995).
- J.R. Miller, "Battery-Capacitor Power Source for Digital
Communication- Simulations Using Advanced Electrochemical Capacitors," Electrochemical
Capacitors, F.M. Delnick and M. Tomkiewicz Editors, PV95-29, pp 246-254, The Electrochemical Society
Proceedings Series, Pennington, NJ (1996).
- C.J. Farahmandi and D. Gideon, "Comparison of Electrochemical
Capacitors and Batteries for Short Duration UPS Applications," Proc. 6th Int. Seminar on Double Layer
Capacitors and Similar Energy Storage Devices, Deerfield Beach, FL (Dec. 9-11, 1996).
- I.N. Varkin, A.D. Klementov, S.V. Litvinenko, N.F. Starodubtsev,
and A.B. Stepanov, "Application of Ultracapacitors as Traction Energy Sources," Proc. 7th Int. Seminar on
Double Layer Capacitors and Similar Energy Storage Devices, Deerfield Beach, FL (Dec. 8-10, 1997).
- A. Nishono, "Development and Current Status of Electric
Double-Layer Capacitors," Ext. Abs.183rd Meeting of the Electrochemical Society,
Honolulu, p. 55 (May 16-21, 1993).
- J.R. Miller, "Technical Status of Large Electrochemical
Capacitors," Proc. Twelfth International Conference on Primary and Secondary Battery Technology and
Applications, Deerfield Beach, FL (March 6-9, 1995).
- J.R. Miller and A.F. Burke, "Electric Vehicle Capacitor Test
Procedures Manual (Revision 0)," Idaho National Engineering
Laboratory Report No. DOE/ID-10491, (October 1994).
- J.R. Miller, "Critical Performance Issues for Electric Vehicle
Load-Leveling Capacitors," Exploratory Research and Development of Batteries for Electric and Hybrid
Vehicles, W.A. Adams, A.R. Landgrebe, and B. Scrosati Editors, PV96-14, pp 16-30, The Electrochemical
Society Proceedings Series, Pennington, NJ (1996).
